Many different processes can be used to produce hydrogen. Thermochemical processes use heat and chemical reactions to release hydrogen from materials such as organic materials (such as fossil fuels and biomass) or water. It is also possible to use electrolysis or solar energy to break down water (H2O) into hydrogen (H2) and oxygen (O2). Microorganisms like bacteria and algae can produce hydrogen through biological processes.
Thermochemical processes
Some thermal processes use energy from various resources (such as natural gas, coal, or biomass) to release hydrogen from their molecular structure. In other processes, heat is combined with a closed chemical cycle to produce hydrogen from raw materials such as water. Learn more about the following thermochemical processes: Methane steam reforming Most of the hydrogen currently produced in the United States is produced by methane steam reforming, a mature production process that uses high-temperature steam (700 ° C to 1000 ° C). sources of methane, such as natural gas. In steam reforming of methane, methane reacts with steam in the presence of a catalyst at a pressure of 3-25 bar (1 bar = 14.5 psig) to produce hydrogen, carbon monoxide, and a small amount of carbon dioxide. carbon. Steam reforming is endothermic. That is, heat must be supplied to the process for it to react. Later, in the so-called “water gas displacement reaction,” carbon monoxide and steam react using a catalyst to produce carbon dioxide and more hydrogen. The last step in the process is called “pressure swing adsorption”, which removes carbon dioxide and other impurities from the gas stream, leaving essentially pure hydrogen. Steam reforming can also be used to produce hydrogen from other fuels such as ethanol, propane and even gasoline. Steam methane reforming reaction CH4 + H2O (+ heat) → CO + 3H2 Water vapour change reaction CO + H2O → CO2 + H2 (+ a small amount of heat) Partial oxidation In partial oxidation, the amount of methane and other hydrocarbons in natural gas Limited oxygen (usually from air) is not enough to oxidize hydrocarbons into carbon dioxide and water completely. Since the available oxygen is less than stoichiometric, the reaction products mainly contain hydrogen and carbon monoxide (and nitrogen if the reaction is carried out with air instead of pure oxygen) and relatively small amounts of carbon dioxide and other compounds. Later, carbon monoxide reacts with water to produce carbon dioxide and more hydrogen in the water-gas exchange reaction. Partial oxidation is an exothermic process: it releases heat. This process is generally much faster than steam reforming and requires a smaller reactor vessel. It can be seen from the partial oxidation chemical reaction that the initial hydrogen production per unit fuel input is less than that obtained by steam reforming the same fuel. Partial oxidation reaction of methane CH4 + ½O2 → CO + 2H2 (+ heat) Water gas exchange reaction CO + H2O → CO2 + H2 (+ small amount of heat) Electrolysis process The electrolysis cell uses electricity to divide the water into hydrogen and oxygen. This technology has been well developed and commercialized, and a system is being developed that can effectively use intermittent renewable energy.
Polymer Electrolyte Membrane Electrolyzer
In a polymer electrolyte membrane (PEM) electrolyzer, the electrolyte is a special solid plastic material. The water reacts at the anode to form oxygen and positively charged hydrogen ions (protons). The electrons flow through the external circuit, and the hydrogen ions are selectively moved to the cathode through the PEM. At the cathode, hydrogen ions combine with electrons from an external circuit to form hydrogen gas. Anode reaction: 2H2O → O2 + 4H + + 4e Cathode reaction: 4H + + 4e → 2H2 Alkaline electrolyzer the working principle of alkaline electrolyzer is to transport hydroxide ions (OH) from the cathode to the anode through the electrolyte, at the cathode Hydrogen is generated on the side. Electrolyzers using liquid alkaline solutions of sodium hydroxide or potassium hydroxide as electrolytes have been on the market for many years. Newer methods using solid alkaline exchange membranes (AEM) as electrolytes show promise on a laboratory scale. Solid Oxide Electrolyzers Solid oxide electrolyzers use solid ceramic materials as electrolytes to selectively conduct negatively charged oxygen (O2) ions at high temperatures to generate hydrogen in a slightly different way. The vapour from the cathode combines with electrons from the external circuit to form hydrogen gas and negatively charged oxygen ions. Oxygen ions pass through the solid ceramic membrane and react at the anode to generate oxygen and generate electrons in external circuits. The solid oxide electrolyzer should operate at a sufficient temperature for the normal operation of the solid oxide film (about 700 ° -800 ° C, compared to the PEM electrolyzer operating temperature of 70 ° -90 ° C, while commercial alkali electrolytic cell temperature is usually below 100 ° C). Advanced laboratory solid oxide electrolyzers based on proton-conducting ceramic electrolytes are expected to lower the operating temperature to 500 ° -600 ° C. The solid oxide electrolyzer can effectively utilize the heat available at these high temperatures (from various sources, including nuclear energy) to reduce the electrical energy required to produce hydrogen from water.
Direct solar water splitting process
The direct solar water splitting or photolysis process uses light energy to split water into hydrogen and oxygen. These processes are currently in several initial stages of research but provide the long-term potential for sustainable hydrogen production with low environmental impact. Learn more about the following solar water splitting processes: PEC’s water splitting processes use semiconductor materials to directly convert solar energy into chemical energy in the form of hydrogen. The semiconductor materials used in the PEC process are similar to those used in photovoltaic solar power generation. Still, for PEC applications, the semiconductor is immersed in a water-based electrolyte, and sunlight provides energy for the decomposition process of the water. See a lab-scale demonstration of this process at the National Renewable Energy Laboratory. The PEC reactor can be constructed as an electrode system or a panel-shaped slurry-based particle system (similar to a photovoltaic panel). Each method has its advantages and challenges. So far, the panel system has received the most extensive research due to the similarity to established photovoltaic panel technology. Thermochemical processes use heat and chemical reactions to release hydrogen from organic materials, such as fossil fuels and biomass, or materials like water. Water (H2O) can also be split into hydrogen (H2) and oxygen (O2) using electrolysis or solar energy. Microorganisms such as bacteria and algae can produce hydrogen through biological processes.
Thermochemical processes
Some thermal processes use energy from various sources (such as natural gas, coal, or biomass) to release hydrogen from their molecular structure. In other methods, heat is combined with a closed chemical cycle to produce hydrogen from raw materials such as water. Learn more about the following thermochemical processes:
Methane steam reforming
Today, most of the hydrogen produced in the United States is made by methane steam reforming, a mature production process that uses high-temperature steam (700 ° C) –1,000 ° C) From a methane source, such as natural gas. In steam reforming of methane, methane reacts with steam in the presence of a catalyst at a pressure of 3 to 25 bar (1 bar = 14.5 psig) to produce hydrogen, carbon monoxide, and a small amount of carbon dioxide. Carbon. Steam reforming is endothermic; that is, heat must be provided to the process for the reaction to proceed.
Subsequently, in the so-called “water gas displacement reaction”, carbon monoxide and vapor are reacted using a catalyst to produce carbon dioxide and more hydrogen. In the final step of the process called “pressure swing adsorption,” carbon dioxide and other impurities are removed from the gas stream, leaving essentially pure hydrogen. Steam reforming can also be used to produce hydrogen from other fuels like ethanol, propane, and even gasoline.
Methane reforming reaction with steam
CH4 + H2O (+ heat) → CO + 3H2
Water gas exchange reaction
CO + H2O → CO2 + H2 (+ small amount of heat)
Partial oxidation
Partial oxidation, methane and other hydrocarbons the reaction of natural gas with a limited amount of oxygen (usually from air) is not enough to completely oxidize hydrocarbons to carbon dioxide and water. Since the available oxygen is minor than stoichiometric, the reaction products contain mainly hydrogen and carbon monoxide (if the reaction is carried out with air instead of pure oxygen, nitrogen) and relatively small amounts of carbon dioxide and other compounds. Later, in the water-gas exchange reaction, carbon monoxide reacts with water to produce carbon dioxide and more hydrogen.
Partial oxidation is an exothermic process: it emits heat. This process is generally much faster than steam reforming and requires a smaller reactor vessel. It can be seen from the partial oxidation chemical reaction that the hydrogen produced per unit of fuel input at the beginning of the process is less than the hydrogen obtained by steam reforming the same fuel.
Partial oxidation of methane
CH4 + ½O2 → CO + 2H2 (+ heat)
Water gas displacement reaction
CO + H2O → CO2 + H2 (+ small amount of heat)
Electrolytic process
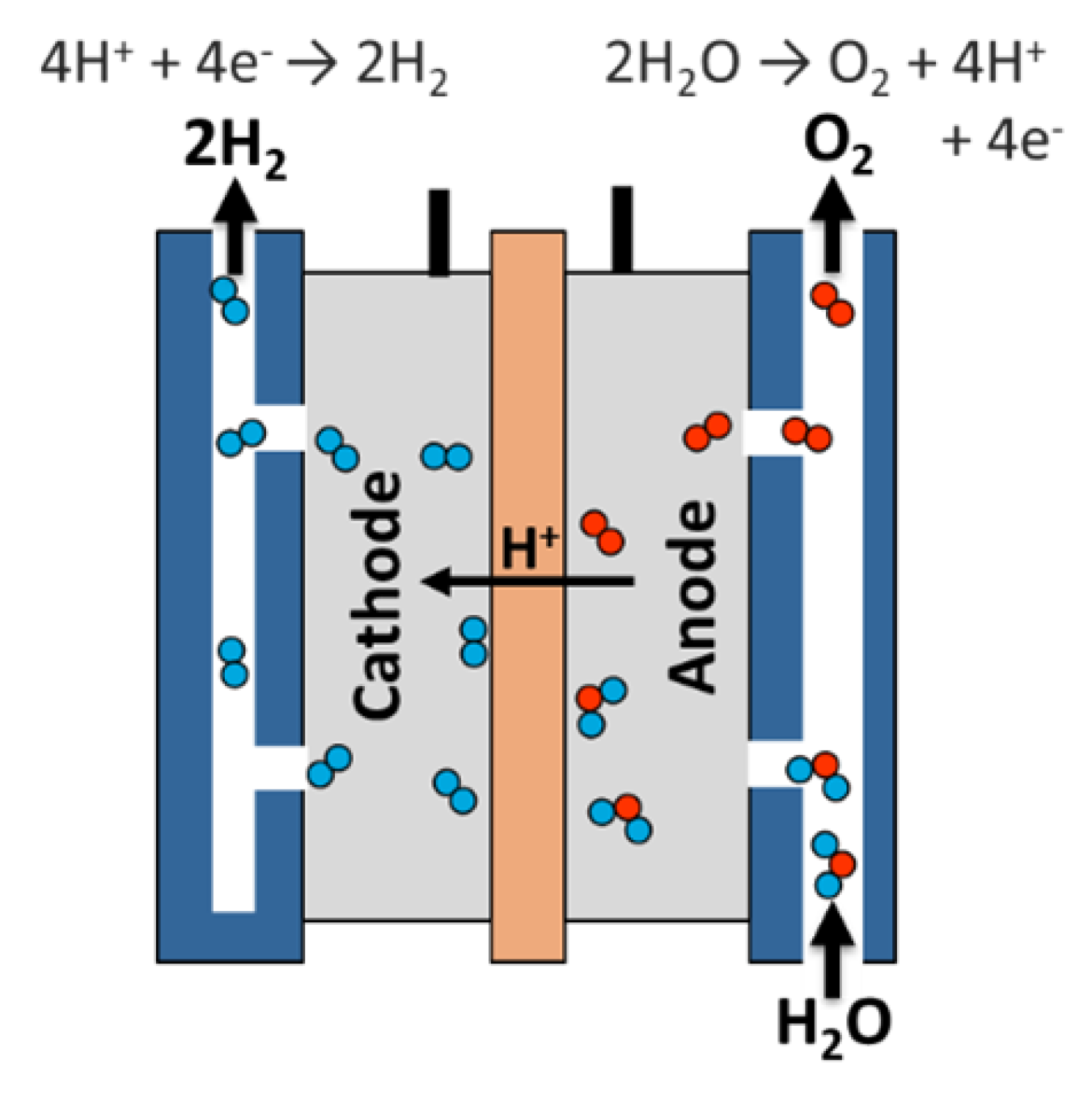
The electrolyte tank uses electricity to divide the water into hydrogen and oxygen. This technology is well developed and commercially available, and systems have been developed that can use intermittent renewable energy efficiently.
Electrolyte polymer Electrolyte membrane
Electrolyte polymer (PEM) Electrolyte is a solid-specific plastic material.
• Water reacts at the anode to form oxygen and positive hydrogen ion (protons).
• Electrons flow through the outer circuit, and hydrogen ions selectively move to the cathode through the PEM.
• In the cathode, the hydrogen ions combine with electrons from the outer circuit to form hydrogen gas. Anodal reaction: 2h 2 or → or 2 + 4h + + 4 h Cathode reaction: 4H + + 4E → 2H2
Alkaline electrolytic cell
Alkaline electrolytic tank
The alkaline electrolytic tank is from the cathode next to the cathode that works from the electrolyte at anode through the hydroxide ion (OH) to the anode. Electrolytic tanks that use liquid alkaline solutions of sodium hydroxide or potassium as the electrolytes are commercially available for many years. A new focus with solid alkali exchange membrane (AEM), since the electrolyte, shows a promising label.
The electrolytic tank of solid oxide
Solid oxide electrolyte is a slightly different shape using solid ceramic material as an electrolyte that selectively conducts negatively charged oxygen ions (or 2) at high temperatures. Generate.
• The cathode vapor is combined with electrons from the outer circuit to form hydrogen gas and negatively charged oxygen ions.
• Oxygen ions pass through the solid ceramic membrane and react with the anode to form an oxygen gas to generate electrons for an external circuit.
Solid oxide electrolytic tanks should operate at a sufficiently high temperature to suitably operate oxide films (PEM electrolyte cells using at 70-90 ° C and commercially available alkaline choices that normally work from about 700 ° C to 800 ° C. It is less than 100 ° C. Advanced Laboscale solid oxide electrolyte based on proton conduction ceramic electrolyte promises a lower operating temperature at 500-600 ° C. Solid oxide electrolytic cells can easily use heat ( From several sources, including nuclear energy) to reduce the amount of electrical power needed to produce water hydrogen.
Direct solar water splitting process
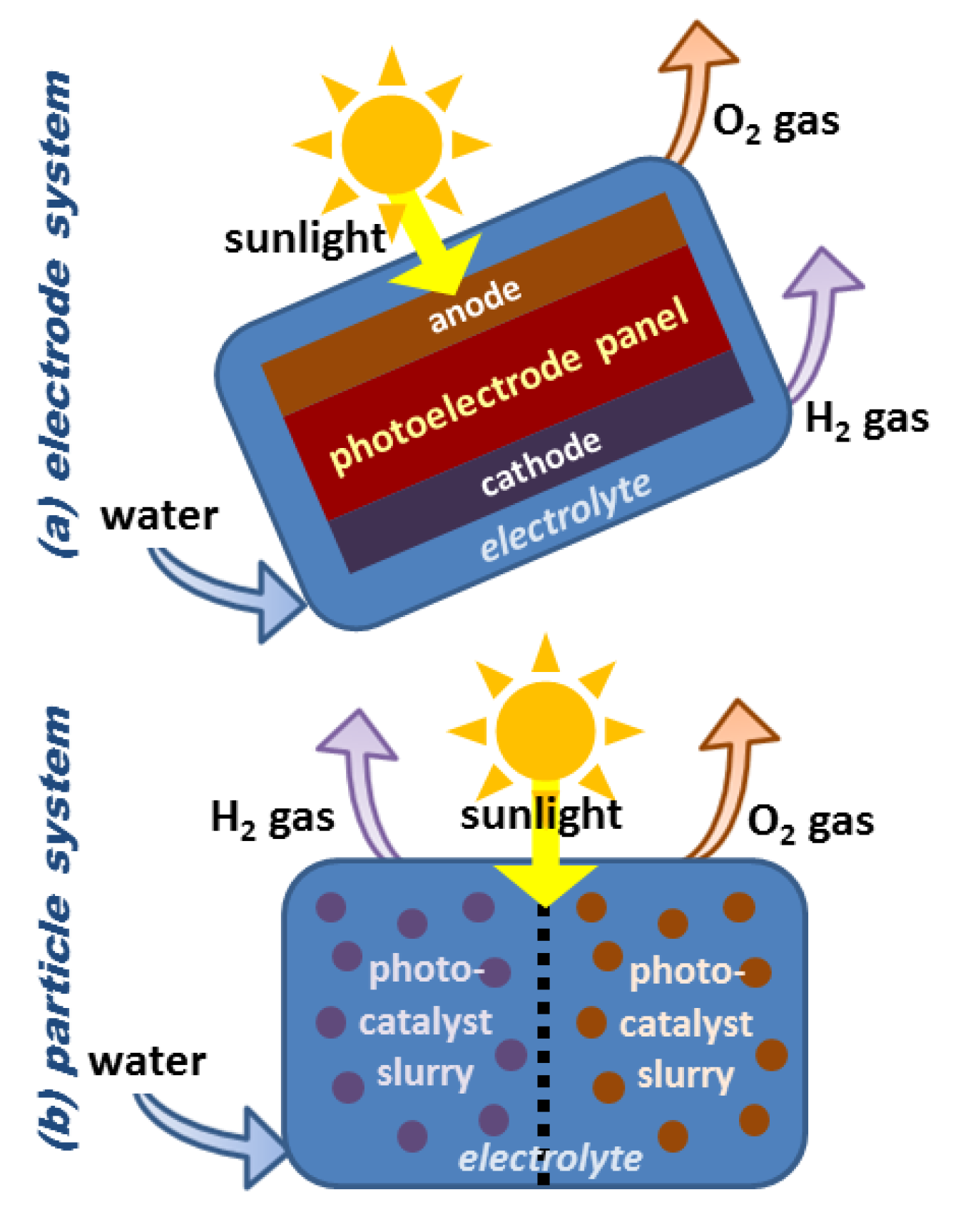
Direct solar water splitting or photolysis process uses light energy to split water into hydrogen and oxygen. These processes are currently in several early stages of research, but they provide the long-term potential for sustainable hydrogen production and have little impact on the environment. Learn more about the following solar water splitting processes:The PEC water splitting process uses semiconductor materials to directly convert solar energy into chemical energy in hydrogen. The semiconductor materials used in the PEC process are similar to those used in photovoltaic solar power generation. Still, for PEC applications, the semiconductor is immersed in a water-based electrolyte and sunlight provides energy for the water decomposition process. View a laboratory-scale demonstration of this process at the National Renewable Energy Laboratory. The PEC reactor can be constructed in a panel (similar to a photovoltaic panel) as an electrode system or a slurry-based particle system. Each method has its advantages and challenges. So far, the panel system has received the most research due to its similarity with the established photovoltaic panel technology. Click on the title of each figure to see some of the different possible implementations of the panel and slurry reactor concepts.
Biological process
Microorganisms such as bacteria and microalgae can use sunlight or organic matter to produce hydrogen through biological reactions. These technological approaches are in the R&D stage and are undergoing pilot demonstrations, but they have the potential for sustainable and low-carbon hydrogen production in the long run.
In fermentation-based systems, microorganisms (such as bacteria) break down organic matter to produce hydrogen. Organic matter can be biomass sources such as refined sugar, corn stalks, or even sewage. Because light is not required, these methods are sometimes referred to as “dark fermentation” methods.
• Microbial biomass conversion
In direct hydrogen fermentation, the microorganisms themselves produce hydrogen. These microorganisms can break down complex molecules in many different ways, and enzymes can combine by-products of some pathways to produce hydrogen. Researchers are studying how to make the fermentation system produce hydrogen faster (higher speed) and have more hydrogen from the same amount of organic matter (higher production). The
Microbial Electrolyzer (MEC) is a device that uses the energy and protons generated by microorganisms to break down organic matter and combines with a small additional current to produce hydrogen. This technology is very new, and researchers are working to improve many aspects of the system, from finding inexpensive materials to identifying the most effective types of microorganisms.